Translate this page into:
Clinical implications of activation of the LIMD1-VHL-HIF1α pathway during head-&-neck squamous cell carcinoma development
For correspondence: Dr Chinmay Kumar Panda, Department of Oncogene Regulation, Chittaranjan National Cancer Institute, Kolkata 700 026, West Bengal, India e-mail: ckpanda.cnci@gmail.com
-
Received: ,
Abstract
Background & objectives
Given the importance of the role of hypoxia induced pathway in different cancers including head-and-neck squamous cell carcinoma (HNSCC), this study delved into elucidating the molecular mechanism of hypoxia-inducible factor-1α (HIF1α) activation in HNSCC. Additionally, it analyzes the alterations of its regulatory genes [von Hippel–Lindau (VHL) and LIM domain containing 1 (LIMD1)] and target gene vascular endothelial growth factor (VEGF) in head-and-neck lesions at different clinical stages in relation with human papillomavirus (HPV) infection.
Methods
Global mRNA expression profiles of HIF1α, VHL, LIMD1 and VEGF were evaluated from public datasets of HNSCC, followed by validation of their expression (mRNA/protein) in an independent set of HPV+ve/–ve HNSCC samples of different clinical stages.
Results
A diverse expression pattern of the HIF1α pathway genes was observed, irrespective of HPV infection, in the datasets. In validation in an independent set of HNSCC samples, high mRNA expressions of HIF1α/VEGF were observed particularly in HPV positive samples. However, VHL/LIMD1 mRNA expression was low in tumours regardless of HPV infection status. In immunohistochemical analysis, high/medium (H/M) expression of HIF1α/VEGF was observed in basal/parabasal layers of normal epithelium, with significantly higher expression in tumours, especially in HPV-positive samples. Conversely, high cytoplasmic VHL expression in these layers gradually decreased with the progression of HNSCC, regardless of HPV infection. A similar trend was noted in LIMD1 expression (nuclear/cytoplasmic) during the disease development. The methylation pattern of VHL and LIMD1 promoters in the basal/parabasal layers of normal epithelium correlated with their expression, exhibiting a gradual increase with the progression of HNSCC. The H/M expression of HIF1α/VEGF proteins and reduced VHL expression was associated with poor clinical outcomes.
Interpretation & conclusions
The results of this study showed differential regulation of the LIMD1-VHL-HIF1α pathway in HPV positive and negative HNSCC samples, illustrating the molecular distinctiveness of these two groups.
Keywords
Basal/parabasal layers
HIF1α regulation
HNSCC-head
and-neck
SCC-squamous cell carcinoma
HPV
normal oral epithelium
prognosis
Head-and-neck squamous cell carcinoma (HNSCC) is one of the most common type of head and neck cancer worldwide and constitutes approximately one-third of all cancer cases from India1. Along with tobacco, differential human papillomavirus (HPV) [high risk(hr)-HPV 16/18] infection rate (0-86.6%), irrespective of anatomical sub-sites, has also been reported to be a major etiological factor2, 6. It appears that recurrent exposure of stem cell-like cells in the basal/parabasal layers of normal oral epithelium to HPV infection or tobacco-associated carcinogens might promote alteration in the intracellular environment, such as oxidative stress response, leading to activation of hypoxia response pathway, which is directly involved in cancer development and progression2,7, 9. The hypoxia response pathway is characterized by the stabilization of hypoxia-inducible factor1α (HIF1α) protein and vascular endothelial growth factor (VEGF) overexpression, plays a prominent oncogenic role in HNSCC development along with various other solid tumours10,11. Escalating over-expression of HIF1α (15-50%) has been noted during HNSCC progression, resulting in adverse prognosis12. Additionally, VEGF, responsible for angiogenesis, has been reported to be overexpressed in 90 per cent of the HNSCC samples, indicating its correlation with disease progression, chemoresistance and poor survival13. It is well reported that Von Hippel–Lindau (VHL) ubiquitinates HIF1α within the nucleus through its interaction with LIMD1 as a bridging protein, followed by cytoplasmic transportation of the protein complex for HIF1α degradation that subsequently results in reduced expression of VEGF11,14,15. Frequent inactivation of LIMD1 and VHL in different sets of HNSCC samples have been reported16,17 along with LIMD1 alterations (mutation, methylation/deletion), reduced mRNA and protein expression18,19. Similarly, loss of heterozygosity (52-67%) at chromosomal location 3p25, residing position of VHL, has been reported in HNSCC20. While null or low expression of VHL was noted in 63 per cent of the tongue cancer samples irrespective of VHL allelic alterations17. Therefore, HIF1α regulation is indisputably a crucial aspect linked to both the development as well as progression of HNSCC. In addition, HPV infection adds scope of alternative molecular features within HNSCC, resulting in heterogeneity within this tumour21. However, the role of the hr-HPV infection in the modulation of HIF1α regulation during HNSCC development has not yet been evaluated in detail.
This study focussed on investigating the comparative expression patterns of HIF1α pathway-associated and regulatory genes in different HNSCC datasets in association with or without HPV infection, followed by validation of the data at gene expression (RNA and protein) and epigenetic level in the independent HNSCC sample set of different clinical stages. It was found that HPV infection shows a distinct effect on the HIF1α regulation in HNSCC samples.
Material & Methods
This study was undertaken at the Department of Oncogene Regulation, Chittaranjan National Cancer Institute (CNCI), Kolkata after appropriate approval of the Institutional Ethical Committee and hospital authorities.
Sample collection
Freshly operated tumour tissues (n=62) and corresponding adjacent normal oral epithelium (n=58) were collected from 62 inpatients from the hospital section of CNCI, Kolkata. The collected tissues were divided to use for (i) immunohistochemical analysis by formalin fixation followed by paraffin embedding, (ii) RNA isolation and (iii) DNA isolation (Supplementary Fig. 1). Tumour grading and staging were done by two independent pathologists and clinicopathological information was collected.
Detection of human papillomavirus (HPV) 16 and 18
HPV infection was detected by polymerase chain reaction (PCR) technique, using MY09 and MY11 primers from the consensus L1 region of virus in the samples. HPV 16/18 typing analysis was done using type-specific primers from the E6 region of HPV 16 and the LCR region of HPV 1822 (Supplementary Table I).
Quantitative analysis of mRNA expression
Total RNA was isolated from primary HNSCC tissue samples (n=30) and their adjacent normal oral tissues (n=30), using TRIzol reagent as manufacturer’s instructions (Invitrogen, USA) (Supplementary Fig. 1). To perform reverse transcription for cDNA preparation, 1 μg of total RNA was used along with Random hexamer (Invitrogen, USA) and M-MuLV-Reverse Transcriptase (Promega, USA)22. Real-time PCR was done using SYBR Green Master Mix (Invitrogen, USA) as described earlier22. Fold expression change (2-ΔΔCT) was used to measure the relative expression of the genes against β2 microglobulin for normalization and was plotted on a log scale23, where ≥0.2 was considered as overexpression, ≤ - 0.2 as underexpression threshold and the range in between as no change (Supplementary Table I and II)
Protein expression analysis by immunohistochemistry
Protein expression of HIF1α, VHL, LIMD1 and VEGF was studied by immunohistochemistry in the adjacent normal oral epithelium (n=58) and the corresponding HNSCC tissues (n=62) (Table I and Supplementary Fig. 1), which were sectioned at 5 μm thickness. The tissue sections (paraffin-embedded/cryosections) of HNSCC as well as the normal adjacent tissue were incubated overnight with primary antibodies against HIF1α (sc-53546), VHL (sc-5575), LIMD1 (mouse monoclonal) and VEGF (sc-507) at a dilution of 1:100 at 4°C. Next, the HRP conjugated secondary antibodies, namely anti-mouse (sc-2005) and anti-rabbit (sc-2004) were used at 1:500 dilution each. 3, 3’ diaminobenzidine (DAB) was used for chromogenic reaction and counterstained with haematoxylin. Slides were photographed under a Bright Field microscope (Leica DM1000, Germany) and evaluation was done according to method followed by Perrone et al24, where two independent observers grade the staining intensity as weak, moderate, or strong along with the percentage of positive cells. The final protein expression was reported as 0-2=low, 3- 5=intermediate, 6-7=high24.
Features | n(%) | HPV 16 | ||
---|---|---|---|---|
+ve, n(%) | –ve, n(%) | P | ||
Total | 62 | 30 (48) | 32 (52) | |
Age, mean±SD | 52±13.37 | |||
Mean≤ | 30 (50) | 17 (57) | 13 (43) | 0.21 |
Mean> | 32 (52) | 13 (41) | 19 (59) | |
Gender | ||||
Male | 39 (63) | 20 (51) | 19 (49) | 0.55 |
Female | 23 (37) | 10 (43) | 13 (57) | |
Primary site | ||||
BM | 28 (45) | 9 (32) | 19 (68) | 0.077 |
TNG | 10 (16) | 5 (50) | 5 (50) | |
ALV | 9 (15) | 5 (56) | 4 (44) | |
Oropharynx | 11 (18) | 7 (64) | 4 (36) | |
Larynx | 4 (6) | 4 (100) | 0 (0) | |
TNM stage | ||||
I | 16 (26) | 9 (56) | 7 (44) | 0.19 |
II | 13 (21) | 7 (54) | 6 (46) | |
III | 17 (27) | 10 (59) | 7 (41) | |
IV | 16 (26) | 4 (25) | 12 (75) | |
Grade | ||||
Mild/WDSCC | 39 (63) | 23 (59) | 16 (41) | 0.09 |
Moderate/MDSCC | 14 (23) | 4 (29) | 10 (71) | |
Poor/PDSCC | 9 (14) | 3 (33) | 6 (67) | |
Node | ||||
+ve | 26 (42) | 16 (62) | 10 (38) | 0.078 |
–ve | 36 (58) | 14 (39) | 22 (61) | |
Tobacco | ||||
+ve | 39 (63) | 20 (51) | 19 (49) | 0.55 |
–ve | 23 (37) | 10 (43) | 13 (57) |
P value represents level of significance during comparison. n, number of samples; BM, buccal mucosa; ALV, alveolus; TNG: tongue; WDSCC, well differentiated squamous cell carcinoma; MDSCC, moderately differentiated squamous cell carcinoma; PDSCC, poorly differentiated squamous cell carcinoma; –ve, factor absent; +ve, factor present; HPV, human papillomavirus; SD, standard deviation; TNM, tumour node metastasis
Microdissection and DNA extraction
(i) Microdissection of the malignant lesions was done for tumour region enrichment (>60%), using surgical knives under a dissecting microscope (Leica MZ16, Germany). The genomic DNA was isolated from the tissues as per routine protocol, described earlier22. The concentration of DNA was measured spectrophotometrically (Supplementary Fig. 1). (ii) The layer-wise promoter methylation analysis was done by using laser capture microscope (LCM, Palm microbeam, Zeiss, Germany) in normal oral epithelium, (n=30), following serial sectioning (paraffin/cryosections) and Haematoxylin-Eosin staining to identify basal/parabasal and spinous layers as described previously22. DNA was isolated from each layer according to the standard procedure22. (Supplementary Fig. 1).
Promoter methylation analysis
In order to study the epigenetic alterations, the promoter methylation analysis of VHL and LIMD1 genes was performed in 30 composite oral epithelium samples, separated basal/parabasal (n=30) and spinous layer (n=30) from the same normal samples as mentioned above and corresponding HNSCC tumour tissues (n=30) as used in the RT-PCR analysis. The promoter methylation status was analyzed by PCR-based methylation-sensitive restriction analysis (MSRA). HpaII (CCGG) restriction enzyme (Promega, Fitchburg, WI) was used to target the CpG‐rich islands in the promoter regions of the genes22. The 445 bp fragment of the β-3A adaptin gene (K1) and the 229 bp fragment of RARβ2 (K2) served as controls for digestion and integrity, respectively22 (Supplementary Table I and II).
Statistical analysis
To determine the risk associated with gene expression and other clinicopathological factors, Fisher’s exact test was applied. All statistical tests used were 2-tailed and a significant level was considered at probability value, P<0.05. Kaplan–Meier method, followed by the Log-rank test, was used to plot survival curves. A multivariate Cox-proportional hazard regression model was used in order to test potential prognostic factors. Hazard ratio was then estimated for each potential prognostic factor with a 95 per cent confidence interval. All calculations were done using software Epi Info 7 (CDC, Atlanta) and IBM SPSS 23 (SPSS, Chicago, IL, USA).
Bioinformatics analysis
The differential mRNA expression pattern of the genes between HPV positive (HPV+ve) and HPV negative (HPV–ve) samples was data mined from HNCDB database25 (http://hncdb.cancerbio.info). Five datasets: TCGA (HNSCC), GSE55542, GSE55544, GSE55546 and GSE39366 were used. The log fold change of the expression in HPV+ve samples with respect to HPV–ve samples was plotted on a heatmap for better visualization and understanding25.
Results
Human papillomavirus (HPV) prevalence in the samples
The HPV infection was seen in 48 per cent (30/62) of the samples irrespective of clinical stages (Table I). Only HPV16 type was identified among the infected samples (Supplementary Fig. 2). Larynx (100%, 4/4) and oropharynx (64%, 7/11) subsites showed maximum infection rate, followed by alveolus (56%, 5/9), tongue (50%, 5/10) and buccal mucosa (32%, 9/28) subsites (Table I). HPV infection frequency was comparable between early-stage (stage I/II) (55%, 16/29) and late-stage (stage III/IV) (42%, 14/33) tumours (P=0.324) (Table I). In the case of tumour grade, node positivity and tobacco usage, HPV infection did not show any significant association (Table I).
Expression profile of the hypoxia-inducible factor-1α (HIF1α) pathway associated genes
Different head-and-neck squamous cell carcinoma (HNSCC) datasets
The comprehensive data mining from the HNCDB database in HPV+ve and HPV–ve HNSCC samples showed a differential expression pattern of HIF1α (log fold change –0.8 to 0.11). Interestingly, significantly low HIF1α expression was noted in HPV+ve samples in three of the datasets (GSE55544, GSE55542 and TCGA) (Fig. 1, Supplementary Fig. 3A and Supplementary Table III). Similarly, significant low expression of VHL (log fold change: –0.07 to 1.55) and LIMD1 (log fold change: –0.61 to –0.48) were evident in HPV+ve samples in three of the datasets (GSE55544, GSE55542, TCGA) and one dataset (GSE39366), respectively (Fig. 1, Supplementary Fig. 3B, C and Supplementary Table III). On the other hand, VEGF (log fold change: –0.19 to 0.33) showed significantly higher mRNA expression in HPV+ve samples in a single dataset (GSE55546) (Fig. 1, Supplementary Fig. 3D and Supplementary Table III).
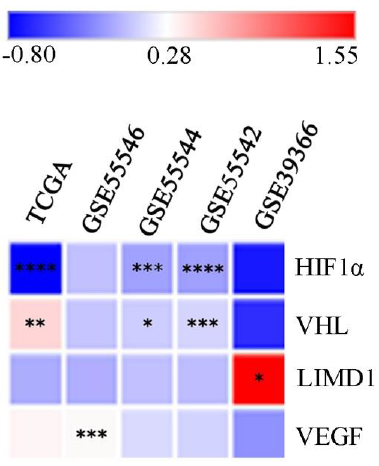
- Global mRNA expression profile of the HIF1α pathway associated genes in HPV+ve/–ve HNSCC samples. The heatmap showing log fold change of expression of the HIF1α pathway associated genes in HPV+ve samples in comparison to HPV–ve HNSCC samples (P*<0.05, **<0.01, ***<0.001, ****<0.0001). HIF1α, hypoxia-inducible factor-1α; HPV, human papilloma virus; HNSCC, head and neck squamous cell carcinoma; VEGF, vascular endothelial growth factor; VHL, von Hippel–Lindau; LIMD1, LIM domain containing 1.
Primary head-and-neck squamous cell carcinoma (HNSCC) samples
Of the HNSCC samples (n=30), overexpression of HIF1α and VEGF with 0.36 and 0.37 median log-fold changes was seen, respectively, with gradual increase with the advancement of the disease (Fig. 2A). However, reduced expression in VHL (-0.3 fold change) and LIMD1 (-0.2 fold change) was noted in the samples with continuous downregulated expression with the disease progression (Fig. 2A). Interestingly, the expression of HIF1α was significantly higher in HPV+ve HNSCC than in the HPV–ve HNSCC samples (P=0.025); whereas VHL (P=0.182), LIMD1 (P=0.083) and VEGF (P=0.493) expression was comparable in between HPV+ve and HPV–ve HNSCC samples (Fig. 2B and Supplementary Table IV).
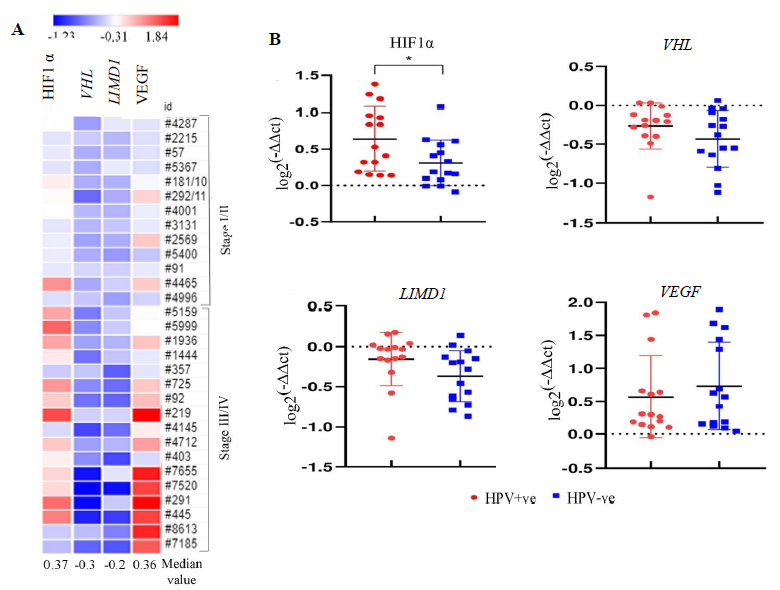
- mRNA expression pattern of HIF1α pathway genes in HNSCC primary tissue samples in comparison to adjacent normal tissue in correlation with HPV infection. (A) The heatmap representing the fold change expression of the genes in each tumour tissue sample in comparison to their paired adjacent normal according to the stage. The median fold change of each gene is also shown. (B) The dot plot representing the comparative analysis of mRNA expression of the HIF1α pathway genes (HIF1α, VHL, LIMD1, VEGF) in HPV+ve/–ve HNSCC samples (P* <0.05).
Expression analysis of HIF1α/LIMD1/ VHL/ VEGF proteins in samples
HIF1α expression profile
HIF1α showed distinct nuclear expression in the tissues. The basal/parabasal expression (43%, 25/58) was significantly higher than the spinous layer (24%, 14/58) (P=0.03) (Fig. 3A and B). The tumours showed substantially higher expression (68%, 42/62) than the basal/parabasal layers (P=0.006) (Fig. 3A, B and Supplementary Table V). Further, the distribution of tumours in early (stage I/II) and late (stage III/IV) stages demonstrated comparable high/medium (H/M) expression pattern in 72 per cent (21/29) and 64 per cent (21/33) of the samples, respectively (Fig. 3B).
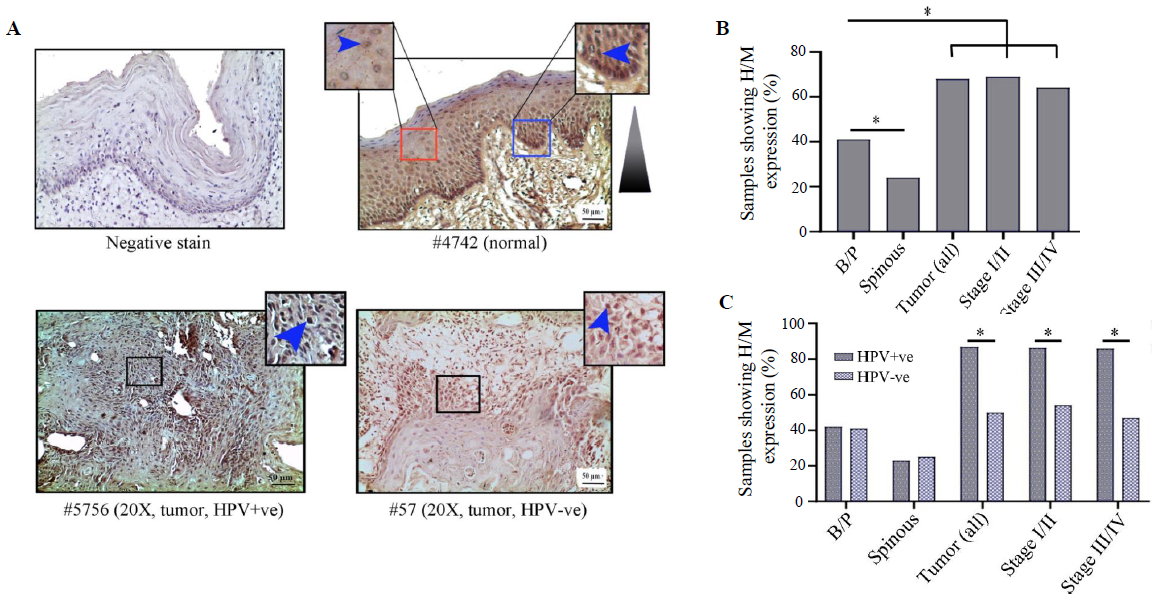
- Immunohistochemical analysis of HIFα protein in HPV+ve/–ve tumours and adjacent normal oral epithelium. (A) Representative image of expression pattern (nuclear) of HIF1α in different layers (B/P and spinous layers) of adjacent normal epithelium and HPV+ve/HPV–ve tumour tissue samples. Inset showing high-power view of the same. (B) The bar graph represents H/M expression of HIF1α in different layers of adjacent normal and tumour tissues of different clinical stages, irrespective of HPV infection status. (C) Bar graph represents HPV infection specific H/M expression pattern of HIF1α in different layers of adjacent normal and tumour tissues of different clinical stages. Blue arrows and blue gradient triangles indicate nuclear expression and nuclear expression pattern, respectively (P*<0.05). B/P, basal/parabasal layers; H/M, high/medium.
The expression profile of HIF1α was comparable in both HPV+ve and HPV–ve basal/parabasal as well as spinous layers of the normal oral epithelium (Supplementary Table V), whereas HPV+ve tumour samples (87%, 26/30) showed significantly higher expression in comparison to the HPV–ve tumour samples (50%, 16/32) (P=0.002) (Fig. 3C and Supplementary Table V). A similar expression pattern was also noted with the progression of the disease in HPV+ve vs. HPV–ve samples, i.e. stage I/II (88% 14/16 vs. 54%, 7/13, P=0.045) and stage III/IV (86%, 12/14 vs. 47%, 9/19, P=0.023), respectively (Fig. 3C).
VHL expression profile
VHL showed differential subcellular expression patterns between basal/parabasal and the spinous layers of normal oral epithelium (Fig. 4A and B). It was noted that the proliferating basal/parabasal layers had predominant high/medium cytoplasmic expression (74%, 43/58) than the nuclear compartment (53%, 31/58) (Fig. 4A, B and Supplementary Table V). Contrastingly, cells in spinous layers showed prevalent high/medium nuclear expression (71%, 44/62) than the cytoplasmic subcellular location (55%, 34/62) (Fig. 4A, B and Supplementary Table V). The signature expression pattern of VHL in the basal/parabasal layers was noted in tumour samples but in significantly lower frequency with 44 per cent (27/62) cytoplasmic (P=0.0006) and 23 per cent (14/62) nuclear expression (P=0.0004) (Fig. 4A, B and Supplementary Table V). It was also noted that the nuclear expression (stage I/II: 21%, 6/29 and stage III/IV: 24%, 8/33) remained comparable (P=0.74), but the cytoplasmic expression (stage I/II: 55%, 16/29 and stage III/IV: 33%, 11/33, P=0.086) of VHL decreased with the progression of the disease (Fig. 4A and B).
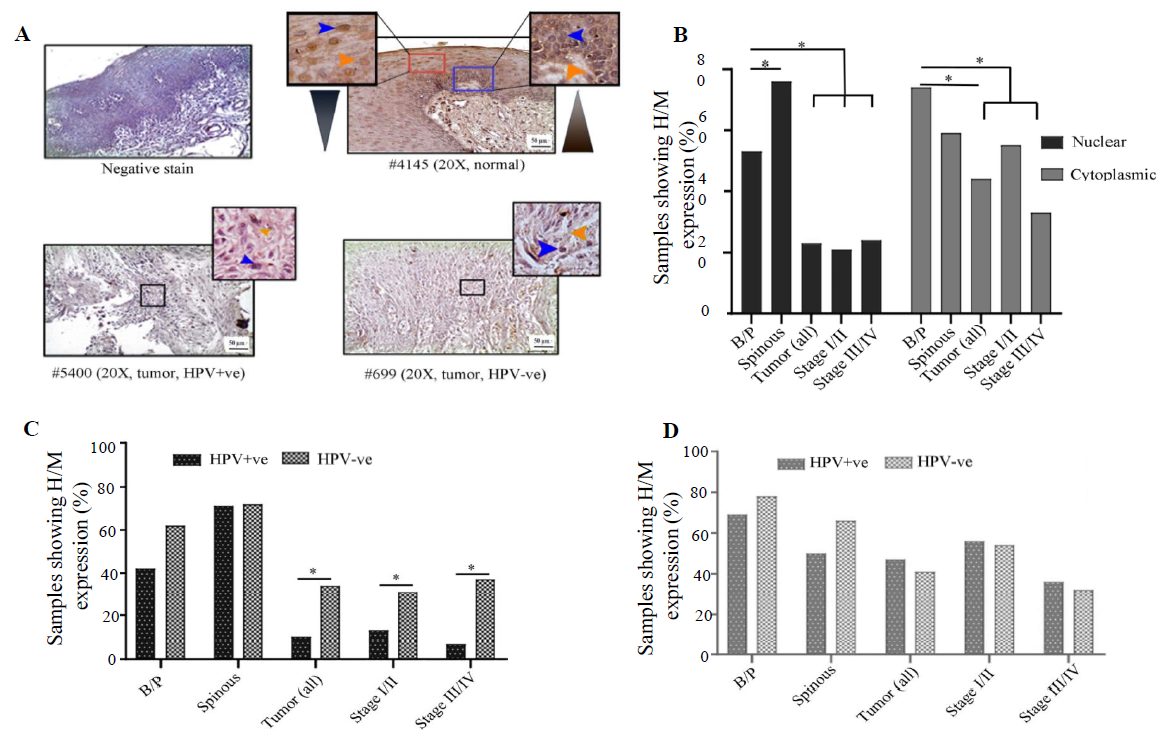
- Immunohistochemical analysis of VHL protein in HPV+ve/–ve tumours and adjacent normal oral epithelium. (A) Representative image of the expression pattern (nuclear/cytoplasmic) of VHL in different layers (B/P and spinous layers) of adjacent normal epithelium and HPV+ve/HPV–ve tumour tissue samples. The insets are showing high-power view of the respective image. (B) Bar graph represents H/M expression of VHL in nuclear and cytoplasmic compartments in different layers of adjacent normal and tumours tissues of different clinical stages, irrespective of HPV infection status. (C and D) The bar graphs represent HPV infection specific comparative expression pattern of VHL in nuclear and cytoplasmic compartments in different layers of adjacent normal and tumours tissues of different clinical stages. Blue and orange arrows indicate nuclear and cytoplasmic expression, respectively and grey gradient triangle indicates nuclear/cytoplasmic expression pattern (P* <0.05).
HPV infection status-specific expression analysis showed that nuclear expression in both HPV+ve and HPV–ve basal/parabasal (HPV+ve: 42%, 11/26 and HPV–ve: 63%, 20/32; P=0.13) and spinous layers (HPV+ve: 70%, 21/26 and HPV–ve: 72%, 23/32; P=0.44) of normal oral epithelium were comparable (Fig. 4C and Supplementary Table V). Similarly, cytoplasmic expression of the basal/parabasal (HPV+ve: 69%, 18/26 and HPV–ve: 78%, 25/32; P=0.45) and spinous layers (HPV+ve: 50%, 13/26 and HPV–ve: 66%, 21/32; P=0.24) were also uniform in both the groups i.e. HPV+ve and HPV–ve (Fig. 4D and Supplementary Table V). In the case of tumour tissues, nuclear expression was significantly low in HPV+ve (10%, 3/30) than the HPV–ve samples (34%, 11/32) (P=0.022) in different clinical stages (Fig. 4C and Supplementary Table V). However, cytoplasmic expression of VHL was comparable between HPV+ve (47%, 14/30) and HPV–ve (41%, 13/32) tumour samples regardless of the progression of the disease (Fig. 4D and Supplementary Table V).
LIMD1 expression profile
In normal oral epithelium uniform nuclear (both basal/parabasal and spinous: 78%, 45/58) and cytoplasmic (basal/parabasal: 62%, 36/58; spinous: 60%, 35/58), the expression of LIMD1 was noted (Fig. 5A, B and Supplementary Table V). In tumours, only 55 per cent (34/62) of the samples showed high/medium nuclear expression, which was significantly low in comparison to basal/parabasal layers (P=0.0084) (Fig. 5A, B and Supplementary Table V). Stage-specific distribution showed comparable high/medium nuclear expression (stage I/II: 59%, 17/29 and stage III/IV: 52%, 17/33; P=0.58) (Fig. 5B). Similarly, only 31 per cent (19/62) of the tumours showed high/medium cytoplasmic expression, which was considerably lower than the cytoplasmic expression of basal/parabasal layers (P=0.018) and the expression pattern also gradually decreased with the progression of the disease (stage I/II: 34%, 10/29 and stage III/IV: 27%, 9/33; P=0.55) (Fig. 5A and B).
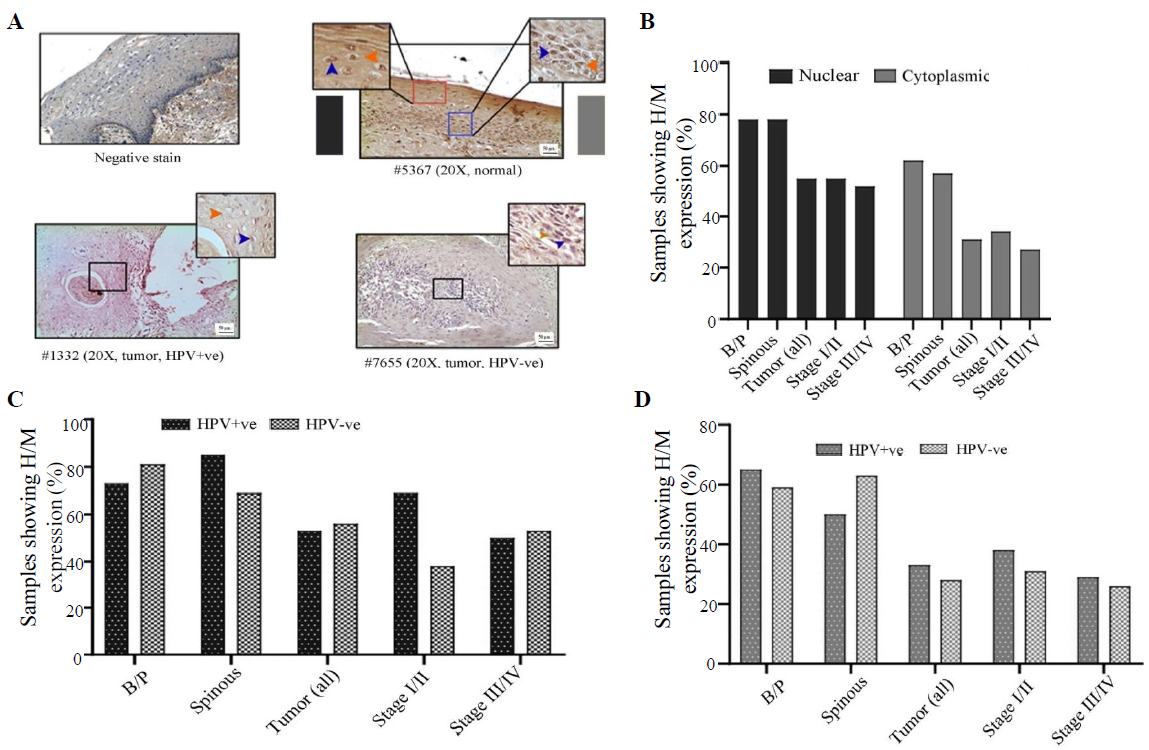
- Immunohistochemical analysis of LIMD1 protein in HPV+ve/–ve tumours and adjacent normal oral epithelium. (A) Representative image of the expression pattern (nuclear/cytoplasmic) of LIMD1 in different layers (B/P and spinous layers) of adjacent normal epithelium and HPV+ve/HPV–ve tumour tissue samples. The insets are showing high-power view of the respective image. (B) The bar graph represents H/M expression of LIMD1 in nuclear and cytoplasmic compartments in different layers of adjacent normal and tumours tissues of different clinical stages, irrespective of HPV infection status. (C and D) The bar graphs represent HPV infection specific comparative expression pattern of LIMD1 in nuclear and cytoplasmic compartments in different layers of adjacent normal and tumours tissues of different clinical stages. Blue and orange arrows indicates nuclear and cytoplasmic expression, respectively and grey gradient rectangle indicates nuclear/cytoplasmic expression pattern.
The expression pattern depending on HPV infection status showed that nuclear expression in basal/parabasal (HPV+ve: 73%, 19/26 and HPV–ve: 81%, 26/32; P=0.47) and spinous layers (HPV+ve: 85%, 22/26 and HPV–ve: 72%, 23/32; P=0.17) of normal oral epithelium were comparable (Fig. 5C, D and Supplementary Table V). Similar result was obtained for the cytoplasmic expression of LIMD1 in basal/parabasal (HPV+ve: 65%, 17/26 and HPV–ve: 59%, 19/32; P=0.65) and spinous layers (HPV+ve: 50%, 13/26 and HPV–ve: 69%, 22/32; P=0.35) (Fig. 5C, D and Supplementary Table V). The nuclear (HPV+ve: 53%, 16/30 and HPV–ve: 56%, 18/32; P=0.82) and cytoplasmic (HPV+ve: 33%, 10/30 and HPV–ve: 28%, 9/32; P=0.66) expression of LIMD1 was also found to be comparable in both HPV+ve and HPV–ve tumour samples (Fig. 5C, D and Supplementary Table V), even when the tumours were distributed according to the stage (stage I/II and stage III/IV) (Fig. 5C and D).
VEGF expression profile
VEGF expression was restricted in cytoplasm in both normal oral epithelium and tumours. It was noted that 43 per cent (25/58) and 24 per cent (14/58) of the samples showed high/medium cytoplasmic expression in basal/parabasal and spinous layers in normal oral epithelium, respectively (P=0.051) (Fig. 6A, B and Supplementary Table V). However, 61 per cent (38/62) of the samples showed high/medium cytoplasmic expression in tumours, which was significantly different from basal/parabasal layers (P=0.04) (Fig. 6A, B and Supplementary Table V). The stage-specific distribution showed 45 per cent (13/29) and 76 per cent (25/33) high/medium expression in stage I/II and stage III/IV tumour samples, respectively (P=0.012) (Fig. 6B).
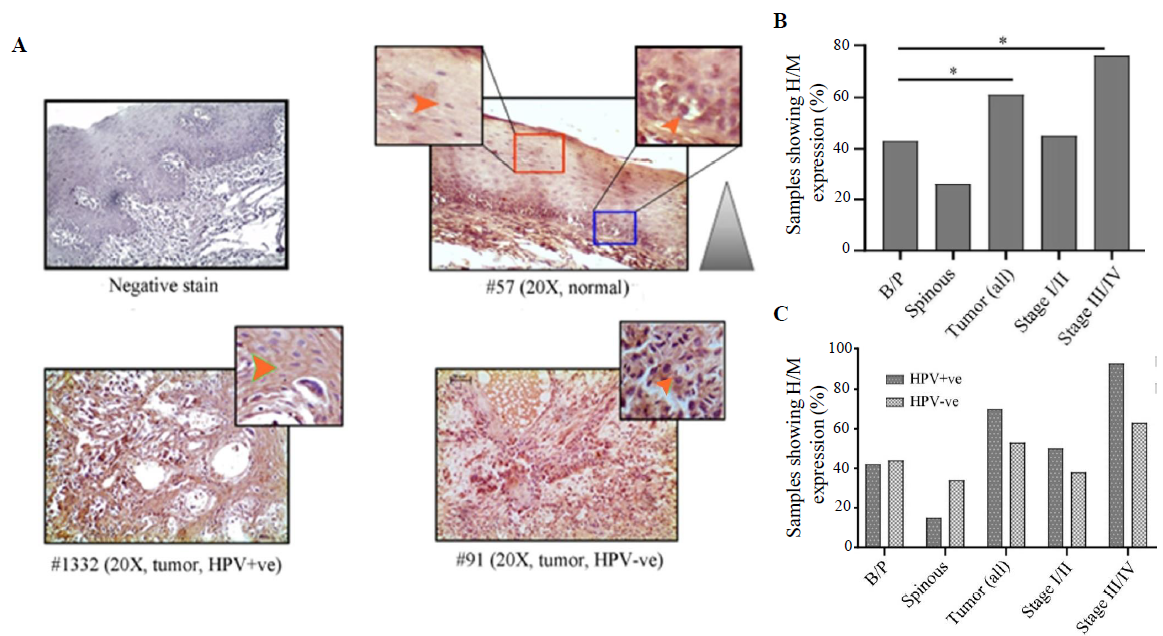
- Immunohistochemical analysis of VEGF protein in HPV+ve/–ve tumours and adjacent normal oral epithelium. (A) Representative image of expression pattern (cytoplasmic) of VEGF in different layers (B/P and spinous layers) of adjacent normal epithelium and HPV+ve/HPV–ve tumour tissue samples. The insets are showing high-power view of the respective image. (B) The bar graph represents H/M expression of VEGF in different layers of adjacent normal and tumour tissues of different clinical stages, irrespective of HPV infection status. (C) The bar graph represents HPV infection specific H/M expression pattern of VEGF in different layers of adjacent normal and tumour tissues of different clinical stages. Orange arrows indicate cytoplasmic expression and grey gradient triangle indicates cytoplasmic expression pattern (P* <0.05).
It was found that HPV+/–ve basal/parabasal (HPV+ve: 42%, 11/26 HPV–ve: 44%, 14/32; P=0.91) and spinous (HPV+ve: 15%, 4/26 HPV–ve: 34%, 11/32; P=0.1) layers of oral epithelium showed comparable cytoplasmic expression (Fig. 6C and Supplementary Table V). In the case of tumours, 70 per cent (21/30) of the HPV+ve samples and 53 per cent (17/32) of the HPV–ve samples showed high/medium VEGF expression (Fig. 6C and Supplementary Table V). However, the difference in the expression pattern between HPV+ve and HPV–ve groups was not significant (P=0.18). However, the expression pattern was distinctly different between HPV+ve (93%, 13/14) and HPV–ve (63%, 12/19) tumours in late-stage (stage III/IV) (P=0.051) than the early-stage (stage I/II) tumours (HPV+ve: 50%, 8/16; HPV–ve: 38%, 5/13; P=0.55) (Fig. 6C).
Association between HIF1α, LIMD1, VHL and VEGF proteins based on expression
It was found that high HIF1α protein expression in the nucleus was significantly correlated with reduced nuclear expression of VHL (r=-0.45, P=0.002) and high expression of VEGF (r=0.037, P=0.003) in tumour samples. However, no such association was noted between the nuclear expression of HIF1α and LIMD1 (r=0.072, P=0.58) in the tumour tissues (Supplementary Fig. 4).
Methylation status of VHL and LIMD1 genes in the samples
In normal oral epithelium, the overall methylation frequency of VHL was seen in 17 per cent (5/30) of the samples (Fig. 7Ai and ii) with a gradual decrease from basal/parabasal (39%, 11/28) to spinous layers (29%, 8/28) (Fig. 7Ai and ii). Like the basal/parabasal layers, high methylation in VHL (60%, 18/30) was seen in the tumours (P=0.12) (Fig. 7Ai and ii). However, the promoter methylation pattern of VHL was comparable in basal/parabasal, spinous and composite normal samples irrespective of HPV infection (Fig. 7A-iii). Similarly, comparable methylation frequencies were also noted in HPV+ve (53%, 8/15) and HPV–ve (67%, 10/15) tumours (P=0.47) (Fig. 7Aiii).
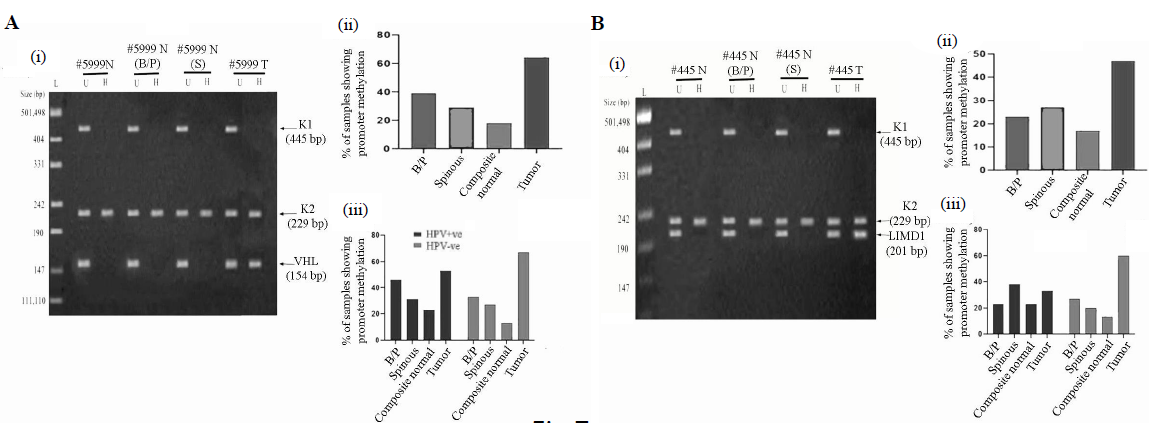
- Promoter methylation analysis of VHL and LIMD1 genes in HPV+ve and HPV–ve samples. (A & B); Panels (i) Representative gel image of promoter methylation analysis using MSRA method for B/P and spinous layers, whole normal (composite) and its corresponding tumour samples. (ii) The bar graph represents the methylation frequency of the genes in different layers of normal oral epithelium, composite normal and corresponding HNSCC tissue samples irrespective of their HPV infection status. (iii) The bar graph represents HPV infection specific methylation frequencies of the genes in the same set of samples. U, undigested samples; H, HpaII digested samples.
In normal oral epithelium, 18 per cent, (5/28) of the samples showed LIMD1 methylation, followed by comparable frequencies of methylation in basal/parabasal (25%, 7/28) and spinous layers (29%, 8/28) (Fig. 7Bi and ii). However, high promoter methylation of LIMD1 (47%, 14/30) was seen in the tumours in comparison to the basal/parabasal layers (P=0.089) (Fig. 7Bi and ii).
In HPV+ve/–ve normal oral epithelium, methylation frequency of LIMD1 did not change significantly (HPV+ve: 23%, 3/13; HPV–ve: 13%, 2/15; P=0.52) (Fig. 7Biii). Similar methylation frequency was also seen in HPV+ve/–ve basal/parabasal (HPV+ve: 23%, 3/13; HPV–ve: 27%, 4/15; P=0.83) and spinous (HPV+ve: 39%, 5/13; HPV–ve: 20%, 3/15; P=0.3) layers (Fig. 7Biii). Interestingly, high promoter methylation of LIMD1 was seen in HPV–ve tumour (60%, 9/15) than HPV+ve samples (33%, 5/15), but was not significant (P=0.15) (Fig. 7Biii).
Clinico-pathological correlation of gene alterations
It was noted that tumour stage was the common potential risk factor associated with disease development as found in both univariate and multivariate analysis (Table II). However, tumour grade and lymph node positivity were found to be potential risk factors only in univariate regression analysis (Table II). HIF1α and VHL expression patterns (nuclear and cytoplasmic) were found to be the prominent risk factors in both univariate and multivariate regression analysis (Table II), whereas VEGF expression was found to be one of the risk factors as per univariate analysis alone (Table II).
Variables | Significant | Exp(B) | 95.0% CI for Exp(B) | |
---|---|---|---|---|
Lower | Upper | |||
A. Univariate Cox regression analysis | ||||
Stage | 0.002 | 0.418 | 0.243 | 0.717 |
HPV infection | 0.222 | 1.398 | 0.817 | 2.391 |
Tobacco usage | 0.464 | 0.817 | 0.476 | 1.404 |
Lymph node | 0 | 2.783 | 1.608 | 4.816 |
Grade | 0.048 | 1.447 | 1.004 | 2.086 |
HIF1α nuclear expression | 0 | 3.154 | 1.694 | 5.873 |
VHL nuclear expression | 0 | 0.119 | 0.048 | 0.294 |
VHL cytoplasmic expression | 0 | 0.176 | 0.095 | 0.328 |
LIMD1 nuclear expression | 0.643 | 0.882 | 0 | 1.502 |
LIMD1 cytoplasmic expression | 0.165 | 0.657 | 0.364 | 1.189 |
VEGF cytoplasmic expression | 0 | 3.657 | 2.036 | 6.568 |
B. Multivariate Cox regression analysis | ||||
Stage | 0 | 0.126 | 0.049 | 0.329 |
HPV infection | 0.378 | 0.733 | 0.367 | 1.462 |
Tobacco usage | 0.241 | 1.566 | 0.739 | 3.316 |
Lymph node | 0.554 | 1.333 | 0.514 | 3.461 |
Grade | 0.767 | 1.079 | 0.653 | 1.783 |
HIF1α nuclear expression | 0 | 6.231 | 2.437 | 15.928 |
VHL nuclear expression | 0 | 0.062 | 0.018 | 0.217 |
VHL cytoplasmic expression | 0.001 | 0.204 | 0.083 | 0.501 |
LIMD1 nuclear expression | 0.784 | 0.906 | 0.448 | 1.832 |
LIMD1 cytoplasmic expression | 0.422 | 1.397 | 0.618 | 3.158 |
VEGF cytoplasmic expression | 0.774 | 1.116 | 0.528 | 2.356 |
Cox proportional hazard regression model to determine prognostic importance of protein expression alterations and different clinico-pathological parameters in patients. B, hazard ratio; CI, confidence interval; HIF1α, hypoxia-inducible factor-1α; VEGF, vascular endothelial growth factor; VHL, von Hippel–Lindau
In survival analysis, high/medium nuclear expression of HIF1α protein, low nuclear/cytoplasmic expression VHL protein expression and high/medium cytoplasmic VEGF expression showed significantly worse prognosis among the HNSCC groups (Supplementary Fig. 5A-C and F). No such association was seen in LIMD1 (nuclear/cytoplasmic) protein expression (Supplementary Fig. 5D and E). Interestingly, high/medium co-expression of HIF1α and VEGF showed poor clinical outcomes (Supplementary Fig. 6A). Moreover, co-alteration in VHL and LIMD1 expression (low nuclear/cytoplasmic) was associated with worst prognosis among the patients (Supplementary Fig. 6B and C).
Discussion
Different studies have reported the clinical significance of HPV infection in HNSCC development as well as progression2, 6.This was also reflected in the present study where the collected samples showed 48 per cent HPV infection frequency with 100 per cent HPV16 infection prevalence.
In the comprehensive data mining approach, it was noted that the genes associated with the HIF1α pathway have differential expression patterns in both HPV+/–ve HNSCC samples. Lower expression patterns of HIF1α and VHL were noted in HPV+ve HNSCC samples in three datasets (GSE55544, GSE55542, TCGA), whereas in majority (4/5) of the datasets, LIMD1 and VEGF showed comparable expression patterns in HPV+/–ve HNSCC samples, indicating that HPV infection might have some role in regulating some of these genes. The bioinformatics data was validated in the collected HNSCC samples, revealing elevated HIF1α and VEGF mRNA expression with disease progression, thereby confirming their oncogenic role11, 13. In accordance with the mRNA expression pattern in the primary HNSCC samples, significantly high HIF1α (nuclear) and VEGF (cytoplasmic) protein expressions were noted in the tumour tissues, which increased parallels with the disease progression similar to prior reports11, 13. In the normal oral epithelium, significantly high HIF1α and VEGF protein expressions were noted in basal/parabasal layers in comparison to the spinous layers, confirming their proactive role in cell proliferation26.
Similar to the mRNA expression, the HIF1α protein expression was significantly higher in HPV+ve than HPV–ve tumours9 and VEGF protein also showed a similar trend. Several studies have suggested that HPV perturb the redox balance27 in the host cell for the viral life cycle and viral DNA integration into the host’s genome28 and thereby activate redox-sensitive pathways resulting in high transcription rate and subsequently high protein expression/stabilization of VEGF and HIF1α in HPV+ve tumour tissues9,29,30. However, comparable HIF1α expression has also been reported in HNSCC, irrespective of HPV infection, opposing the interpretation of the direct association between HIF1α/VEGF and HPV infection in head-and-neck malignancy31,32. Although the HIF1α/VEGF transcription/translation rate was lower in HPV–ve HNSCC samples than in HPV+ve tumour tissues, it remained higher than in normal epithelium. This could be attributed to the intrinsic cellular response to hypoxia induced in solid tumour33.
Contrastingly, LIMD1 and VHL tumour suppressive role was authenticated with a gradual decrease in mRNA expression in advanced tumour stages. These mRNA data were further validated by the protein expression, showing lower expression of both VHL and LIMD1 protein in the tumour tissues16,17. It was interesting to note that VHL protein showed preferential cytoplasmic expression in basal/parabasal than the spinous layers of normal oral epithelium and also in tumour tissues. Previously it was reported that VHL remains sequestered in cytoplasm in proliferating cells as it interferes with the assembly of transcriptionally active Elongin complexes and displaces Elongin A, inhibiting the transcription of the cell proliferation associated genes34,35. This might be the reason for the differential nucleo-cytoplasmic localization of VHL in proliferating basal/parabasal layers and non-proliferating mature spinous layers of normal oral epithelium and also in the tumour tissues34, 37. However, both the nuclear and the cytoplasmic expression of VHL gradually reduced in tumour tissues with the progression of the disease, indicating its overall tumour suppressive role during HNSCC development38, 40.
The VHL and LIMD1 mRNA expression was comparable in both HPV+ve and HPV–ve tumour samples. When we classified the protein expression pattern of VHL in normal epithelium in association with HPV infection, it was found that VHL nucleo-cytoplasmic expression was similar in both the layers of normal epithelium irrespective of HPV infection status, confirming insignificant influence of HPV on VHL expression in normal tissues. Interestingly, in tumour tissues, the nuclear expression was found to be significantly lower in the HPV+ve group than HPV–ve, especially in the advanced stages. However, this statement needs to be authorized by further studies. Studies have reported that viruses exploit the host’s ubiquitinoylation machinery and reprogramme the substrate specificity, leading to cellular transformation41,42. It was documented that HPV oncoprotein E7 replaces VHL from the E3 ubiquitin ligase complex and heavily ubiquitinates VHL itself, reducing its nuclear localization and stability in HPV+ve tumour tissues, resulting in high HIF1α and VEGF expression43, 45. However, this statement needs further verification by confirming protein-protein interaction by co-immunoprecipitation in both HPV+ve and HPV–ve cell lines. In the HPV–ve tumour tissue, low VHL expression (nuclear/cytoplasmic) was also noted, which could be explained by frequent genetic/epigenetic alteration of VHL in HNSCC as reported by several other studies17,20. The LIMD1 protein showed relative expression in both HPV+ve and HPV–ve tumour samples, corroborating its HPV infection-independent downregulated expression during disease development18,19.
It is important to mention that discrepancies in the mRNA expression pattern of genes between some of the datasets and our HNSCC samples might be due to differences in the methodology used in the analysis and etiological factors associated with the disease.
The oncogenic role of HIF1α showed a significant positive association with VEGF in HNSCC tissues like other cancers46,47. Therefore, assessment of the HIF1α and VEGF co-operative expression pattern in malignant samples could be used as predictive markers to analyze the tumour behaviour, important for selecting suitable targeted therapy48, 50. However, a report is also available pointing to no significant association between these two proteins in oral squamous cell carcinoma51. The agnostic role of VHL against HIF1α was validated by their significant negative correlation in the primary HNSCC tissue samples, conceivably indicating potentiality of VHL as therapeutic candidate. Alternatively, the insignificant association of HIF1α with LIMD1 was noted in HNSCC samples, implying LIMD1 independent HIF1α regulation52 while, several studies have also validated the crucial role of LIMD1 in HIF1α stabilization53.
The promoter methylation pattern of VHL and LIMD1 was consistent with their mRNA/protein expression data, indicating methylation as one of the molecular mechanisms for the VHL and LIMD1 reduced expression in tumour tissues19. The VHL showed comparable methylation frequency in both HPV+ve and HPV–ve tumour tissues, but the methylation percentage of LIMD1 was higher in HPV–ve as compared to HPV+ve tumours. Although the difference in methylation frequency between HPV+ve and HPV–ve samples was not significant, it suggested a trend among samples with a probable distinct molecular event for LIMD1 in HPV–ve tumour. It is noteworthy that several studies have also reported allelic deletion along with methylation in both VHL and LIMD1 in HNSCC samples resulting in their lower expression in tumour tissues16, 20. We also did the layer-wise methylation analysis for both VHL and LIMD1 in normal oral epithelium, but no significant difference was noted, even in the presence or absence of HPV infection.
Finally, the risk analysis and survivability study showed that tumour stage, HIF1α, VEGF and VHL expression patterns in different subcellular pockets play an imperative role in HNSCC, indicating their potentiality as the key regulatory factors. It is important to highlight that high/medium expression of HIF1α and its target gene VEGF are associated with poor patient prognosis as these might contribute to tumour metastasis and further aggressiveness of the disease. While under expression of VHL in both nuclear and cytoplasmic locations facilitate HIF1α stability and might be responsible for poor patient outcomes. These data were further validated with the poor patient survivability in tumour samples showing high/medium co-expression of HIF1α–VEGF or low co-expression of VHL-LIMD1 (nuclear/cytoplasmic).
Several HIF1α, VEGF and VHL targetable therapeutic molecules have been under clinical trial for HNSCC treatment, but none has so far received a concession for clinical use in HNSCC patients. This study features the distinct molecular profile of HIF1α and its associated/regulatory genes in HNSCC; moreover, it also focuses on the comparative discrete expression pattern of these genes in HPV+ve and HPV–ve HNSCC among Indians. This study offers information related to prognosis and clinical strategies to opt for combinatorial therapeutic intervention along with the existing strategies depending on the HPV-specific disease condition.
Acknowledgment
The authors acknowledge the receipt of primary antibody –LIMD1(mouse monoclonal) as a gift from Dr. Tyson V. Sharp, Barts Cancer Institute, Queen Mary University of London.
Financial support & sponsorship
This study received financial support from Department of Science and Technology (Govt. of India), Kiran Division (SR/WOS-A/LS-278/2016). Author BC received UGC-NET Fellowship (Sr. No. 2121430433, Ref. No.: 21/12 / 2014) & EU-V dated 08.06.2015. Author CKP received NASI Senior Scientist Platinum Jubilee Fellowship (2020).
Conflicts of Interest
None.
Use of Artificial Intelligence (AI)-Assisted Technology for manuscript preparation
The authors confirm that there was no use of AI-assisted technology for assisting in the writing of the manuscript and no images were manipulated using AI.
References
- Head and neck squamous cell carcinoma in young adults: A hospital-based study. Indian J Med Paediatr Oncol. 2019;40:18-22.
- [Google Scholar]
- Human papillomavirus in head and neck cancer in India: Current status and consensus recommendations. South Asian J Cancer. 2017;6:93-8.
- [CrossRef] [PubMed] [PubMed Central] [Google Scholar]
- Human papillomaviruses in 91 oral cancers from Indian betel quid chewers-high prevalence and multiplicity of infections. Int J Cancer. 1995;61:450-4.
- [CrossRef] [PubMed] [Google Scholar]
- Identification of human papillomaviruses in tumors of the oral cavity in an Indian community. Int J Cancer. 2005;113:946-50.
- [CrossRef] [PubMed] [Google Scholar]
- Prevalence of high-risk human papillomavirus type 16 and 18 in oral and cervical cancers in population from Gujarat, West India. J Oral Pathol Med. 2014;43:293-7.
- [CrossRef] [PubMed] [Google Scholar]
- The prevalence and clinicopathological correlation of human papillomavirus in head and neck squamous cell carcinoma in India: A systematic review article. Cancer Treat Res Commun. 2021;26:100301.
- [CrossRef] [PubMed] [Google Scholar]
- When Oxidative Stress Meets Epigenetics: Implications in Cancer Development. Antioxidants (Basel). 2020;9:468.
- [CrossRef] [PubMed] [PubMed Central] [Google Scholar]
- Hypoxia and free radicals: role in tumor progression and the use of engineering-based platforms to address these relationships. Free radi biol med. 2015;79:281-91.
- [CrossRef] [Google Scholar]
- Oxidative stress and HPV carcinogenesis. Viruses. 2013;5:708-31.
- [CrossRef] [PubMed] [PubMed Central] [Google Scholar]
- HIF-1α pathway: role, regulation and intervention for cancer therapy. Acta Pharm Sin B. 2015;5:378-89.
- [CrossRef] [PubMed] [PubMed Central] [Google Scholar]
- Head and neck squamous cell carcinoma. Nat Rev Dis Primers. 2020;6:92.
- [CrossRef] [PubMed] [PubMed Central] [Google Scholar]
- Overexpression of hypoxia-inducible-factor 1α(HIF-1α) in oesophageal squamous cell carcinoma correlates with lymph node metastasis and pathologic stage. Bri J cancer. 2003;89:1042-7.
- [Google Scholar]
- An update on angiogenesis targeting in head and neck squamous cell carcinoma. Cancers Head Neck. 2020;5:5.
- [CrossRef] [PubMed] [PubMed Central] [Google Scholar]
- Oxygen-dependent ubiquitination and degradation of hypoxia-inducible factor requires nuclear-cytoplasmic trafficking of the von Hippel-Lindau tumor suppressor protein. Mol Cell Biol. 2002;22:5319-36.
- [CrossRef] [PubMed] [PubMed Central] [Google Scholar]
- The LIMD1 protein bridges an association between the prolyl hydroxylases and VHL to repress HIF-1 activity. Nat Cell Biol. 2012;14:201-8.
- [CrossRef] [PubMed] [Google Scholar]
- Loss of heterozygosity on chromosomes 3p,8p,9p and 17p in the progression of squamous cell carcinoma of the larynx. J Korean med sci. 2004;19:345-51.
- [CrossRef] [PubMed] [Google Scholar]
- Expression of von Hippel-Lindau tumor suppressor protein (pVHL) characteristic of tongue cancer and proliferative lesions in tongue epithelium. BMC cancer. 2017;17:381.
- [CrossRef] [PubMed] [PubMed Central] [Google Scholar]
- LIMD1 is more frequently altered than RB1 in head and neck squamous cell carcinoma: clinical and prognostic implications. Mol cancer. 2010;9:58.
- [CrossRef] [PubMed] [PubMed Central] [Google Scholar]
- Differential transmission of the molecular signature of RBSP3, LIMD1 and CDC25A in basal/ parabasal versus spinous of normal epithelium during head and neck tumorigenesis: A mechanistic study. PLoS One. 2018;13:e0195937.
- [CrossRef] [PubMed] [PubMed Central] [Google Scholar]
- Allelic loss at chromosomes 3p, 8p, 13q, and 17p associated with poor prognosis in head and neck cancer. J Natl Cancer Inst. 1994;86:1524-9.
- [CrossRef] [PubMed] [Google Scholar]
- Molecular Tumor Subtypes of HPV-Positive Head and Neck Cancers: Biological Characteristics and Implications for Clinical Outcomes. Cancers. Basel. 2021;13:2721.
- [Google Scholar]
- Differential Wnt-β- catenin pathway activation in HPV positive and negative oral epithelium is transmitted during head and neck tumorigenesis: clinical implications. Med Microbiol Immunol. 2021;210:49-63.
- [CrossRef] [PubMed] [PubMed Central] [Google Scholar]
- Analysis of Relative Gene Expression Data Using Real-Time Quantitative PCR and the 2−ΔΔCT Method. Methods. 2001;25:402-8.
- [CrossRef] [PubMed] [Google Scholar]
- Molecular and Cytogenetic Subgroups of Oropharyngeal Squamous Cell Carcinoma. Clin Cancer Res. 2006;12:6643-51.
- [CrossRef] [PubMed] [Google Scholar]
- HNCDB: An Integrated Gene and Drug Database for Head and Neck Cancer. Front Oncol. 2019;9:371.
- [CrossRef] [PubMed] [PubMed Central] [Google Scholar]
- Deregulation of LIMD1-VHL-HIF-1α-VEGF pathway is associated with different stages of cervical cancer. Biochem J. 2018;475:1793-806.
- [CrossRef] [PubMed] [Google Scholar]
- Oxidative stress and HPV carcinogenesis. Viruses. 2013;5:708-31.
- [CrossRef] [PubMed] [PubMed Central] [Google Scholar]
- Cellular redox, cancer and human papillomavirus. Virus Res. 2018;246:35-45.
- [CrossRef] [PubMed] [Google Scholar]
- Hypoxia-inducible factor-1α activation in HPV-positive head and neck squamous cell carcinoma cell lines. Oncotarget. 2017;8:89681-91.
- [CrossRef] [PubMed] [PubMed Central] [Google Scholar]
- Redox-sensitive signalling pathways regulated by human papillomavirus in HPV-related cancers. Rev Med Virol. 2021;31:e2230.
- [CrossRef] [PubMed] [Google Scholar]
- The prognostic significance of hypoxia inducing factor 1-α in oropharyngeal cancer in relation to human papillomavirus status. Oral Oncol. 2013;49:354-9.
- [CrossRef] [PubMed] [Google Scholar]
- HPV, hypoxia and radiation response in head and neck cancer. Br J Radiol. 2019;92:20180047.
- [CrossRef] [PubMed] [PubMed Central] [Google Scholar]
- Hypoxia-inducible factors HIF-1alpha and HIF-2alpha in head and neck cancer: relationship to tumor biology and treatment outcome in surgically resected patients. Cancer Res. 2002;62:2493-7.
- [PubMed] [Google Scholar]
- Subcellular localization of the von Hippel-Lindau disease gene product is cell cycle-dependent. Int J Cancer. 1998;78:62-9.
- [CrossRef] [PubMed] [Google Scholar]
- Nuclear/cytoplasmic localization of the von Hippel-Lindau tumor suppressor gene product is determined by cell density. Pro Nat Acad Sci USA. 1996;93:1770-5.
- [CrossRef] [Google Scholar]
- Endoplasmic reticulum/cytosolic localization of von Hippel-Lindau gene products is mediated by a 64-amino acid region. Int J Cancer. 2001;91:457-67.
- [CrossRef] [PubMed] [Google Scholar]
- Role of nuclear and cytoplasmic localization in the tumour-suppressor activity of the von Hippel-Lindau protein. Oncogene. 2003;22:3992-7.
- [CrossRef] [PubMed] [Google Scholar]
- Regulation of hypoxia-inducible mRNAs by the von Hippel-Lindau tumor suppressor protein requires binding to complexes containing elongins B/C and Cul2. Mol Cell Biol. 1998;18:732-41.
- [CrossRef] [PubMed] [PubMed Central] [Google Scholar]
- Transcription-dependent nuclear-cytoplasmic trafficking is required for the function of the von Hippel-Lindau tumor suppressor protein. Mol Cell Biol. 1999;19:1486-97.
- [CrossRef] [PubMed] [PubMed Central] [Google Scholar]
- Head and neck paragangliomas in von Hippel-Lindau disease and multiple endocrine neoplasia type 2. J Clin Endocrinol Metab. 2009;94:1938-44.
- [CrossRef] [PubMed] [PubMed Central] [Google Scholar]
- Viral Takeover of the Host Ubiquitin System. Front Microbiol. 2011;2:161.
- [CrossRef] [PubMed] [PubMed Central] [Google Scholar]
- Ubiquitination, ubiquitin-like modifiers, and deubiquitination in viral infection. Cell Host & Microbe. 2009;5:559-70.
- [CrossRef] [PubMed] [Google Scholar]
- Human papillomavirus type 16 E7 oncoprotein associates with the cullin 2 ubiquitin ligase complex, which contributes to degradation of the retinoblastoma tumor suppressor. J Virol. 2007;81:9737-47.
- [CrossRef] [PubMed] [PubMed Central] [Google Scholar]
- BC-box protein domain-related mechanism for VHL protein degradation. Pro Nat Acad Sci. 2013;110:18168-73.
- [CrossRef] [Google Scholar]
- Retraction: Ubiquitin/SUMO modification regulates VHL protein stability and nucleocytoplasmic localization. PLoS One. 2022;17:e0265155.
- [CrossRef] [PubMed] [PubMed Central] [Google Scholar]
- Molecular mechanism of HIF-1-independent VEGF expression in a hepatocellular carcinoma cell line. Int J Mol Med. 2011;28:449-54.
- [CrossRef] [PubMed] [Google Scholar]
- Hypoxia-Inducible Factor1-Α (HIF1α) and Vascular Endothelial Growth Factor-A (VEGF-A) Expression in De Novo AML Patients. Asian Pac J Cancer Prev. 2019;20:705-10.
- [CrossRef] [PubMed] [PubMed Central] [Google Scholar]
- Therapeutic Targeting Hypoxia-Inducible Factor (HIF-1) in Cancer: Cutting Gordian Knot of Cancer Cell Metabolism. Front Genet. 2022;13:849040.
- [CrossRef] [PubMed] [PubMed Central] [Google Scholar]
- Therapeutic approaches for the treatment of head and neck squamous cell carcinoma-An update on clinical trials. Transl Oncol. 2022;21:101426.
- [CrossRef] [PubMed] [PubMed Central] [Google Scholar]
- Angiogenesis Inhibitors for Head and Neck Squamous Cell Carcinoma Treatment: Is There Still Hope? Front Oncol. 2021;11:683570.
- [CrossRef] [PubMed] [PubMed Central] [Google Scholar]
- The correlation between HIF-1 alpha and VEGF in oral squamous cell carcinomas: Expression patterns and quantitative immunohistochemical analysis. J Chin Med Assoc. 2018;81:370-5.
- [CrossRef] [PubMed] [Google Scholar]
- The chaperone-dependent ubiquitin ligase CHIP targets HIF-1α for degradation in the presence of methylglyoxal. PLoS One. 2010;5:e15062.
- [CrossRef] [PubMed] [PubMed Central] [Google Scholar]
- A HIF-LIMD1 negative feedback mechanism mitigates the pro-tumorigenic effects of hypoxia. EMBO Mol Med. 2018;10:e8304.
- [CrossRef] [PubMed] [PubMed Central] [Google Scholar]